-
Photoreforming
-
CO2 Utilisation
-
Bio-hybrids
Why make oxygen when you could make useful chemicals
instead?
Solar-driven fuel synthesis is a sustainable and potentially
economical technology for producing energy carriers such as
“green” H2 fuel through water splitting. Photocatalytic
water splitting processes are usually limited by the water
oxidation half-reaction, which is kinetically and energetically
demanding as well as requires often expensive catalysts and
unsustainable sacrificial reagents. Our research aims to overcome
these challenges by using alternative oxidation half-reactions to
drive the breakdown of waste polymers or chemicals into valuable
organic products. We utilise a variety of novel photocatalysts –
including quantum dots and carbon-based nanomaterials – to (1)
develop light-driven, high-yield organic transformations and (2)
“photoreform” plastic- or biomass-derived waste into organics and
fuel. Our aim is to enhance the sustainability and economic value
of solar fuels by developing processes that simultaneously produce
fuels and drive value-added organic transformations.
Selected Publications
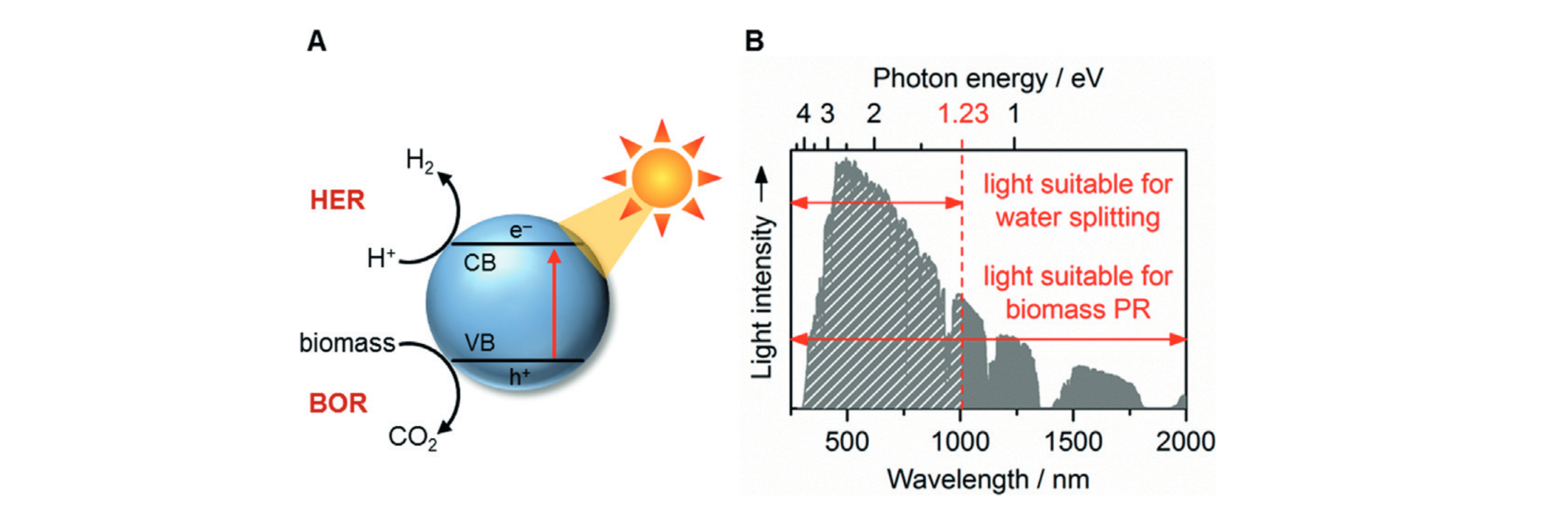
Solar hydrogen generation from lignocellulose.
Kuehnel,M. F.; Reisner, E. Angew. Chem. Int. Ed., 2018, 57, 3290-3296.
Why release CO2 into the atmosphere if you could
use it to make fuels and chemicals?
The sustainable utilisation of the greenhouse gas CO2
represents a key step towards accomplishing a circular carbon
economy. To address this goal, we interface light absorbers with
suitable catalysts for the light-driven conversion of CO2
to value-added chemicals, including CO, formate, methane, or
liquid multicarbon products. Our research covers various facets of
CO2 conversion, from fundamental studies on
electrocatalytic surface-bound interactions, to applied research
on device integration and upscaling. Molecular catalysts are
immobilised onto nanostructured metal oxide, lead halide
perovskite, and silicon semiconductors to promote highly-selective
CO2 conversion in both aqueous and organic media.
Spectroelectrochemical studies on those (photo)electrodes uncover
mechanistic insights into optimal catalyst loading and
selectivity. Synthetic catalysts are functionalised with a variety
of anchor groups to enable photocatalysis in colloidal systems
involving quantum dot, carbon nitride and carbon dot
nanoparticles. Photoelectrochemical “artificial leaf” devices and
particulate photocatalyst sheets are being developed to probe the
stability and scalability of our systems, taking practical aspects
as variable daylight conditions and day-night cycles into account.
Overall, our efforts strive towards establishing solar carbon
fuels as a competitive alternative to fossil fuels in the future.
Selected Publications
Why not combine the best of materials science and biology
to develop new concepts for solar energy conversion?
Semi-artificial photosynthetic systems aim to overcome the
limitations of natural and artificial photosynthesis while
providing an opportunity to investigate their respective
functionality. Enzymes are macromolecular biological catalysts
that have been naturally selected over billions of years to
perform specific reactions with high selectivity and efficiency.
In particular, we are interested in interfacing photosynthetic and
redox active enzymes with custom-made high surface area electrodes
to study their fundamental biology and drive interesting
endergonic reactions. In parallel, we examine how more complex
living microorganism systems can be recruited for in vivo fuel and
chemical production.
Our lab employs a suite of chemical biology and biophysical
methods, including advanced (photo)electrochemical techniques such
as rotating ring disk electrochemistry, resonance Raman and
infrared spectroscopy and quartz crystal microbalance
measurements. To develop enzyme and cell-based hybrid
(photo)electrochemical devices with light absorbing semiconductors
such as metal oxides, perovskites and silicon we design
high-surface area electrode materials, such as metal oxides,
carbon nanotubes and graphene as conductive supports with high
loading. We also study photocatalytic systems with semiconducting
nanoparticles such as carbon dots, graphitic carbon nitride and
quantum dots for hybrid solar fuel generation in suspension.